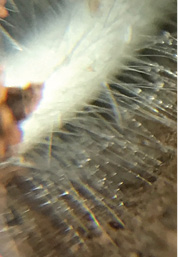
Article Written By: Nicole Masters
Most humans feel adept and evolved in their ability to communicate their needs, wants, and desires. However, our communication skills pale in comparison to plants and the microbiology in the rhizosphere. Imagine being a plant, rooted into the earth and unable to flee from a predator or undesirable situation. Imagine being a tiny microbe, down in the soil, away from light, in very hostile environments. Both plants and microbes have incredible sensory and communication systems that help them determine and distinguish between food, friend, and foe. This article will highlight some of the systems and will hopefully whet your appetite to take a deeper dive into the subject!
If you zoom into a plant cell, you will see that every single cell wall has hundreds of thousands of receptors ready to bind to signaling molecules. These receptors function like keys, turning gene expression on or off, which affect how a cell functions without allowing molecules to enter the cell.
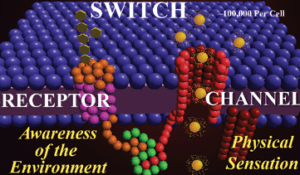
Photo By: Bruce Lipton
This switching on and off of genes can influence cell metabolism, biogenesis, growth, group behaviors, and responses to nutrient, energy, hormonal, pathogens, and environmental cues.
The number and nature of the understandings around plant and biological “infochemicals” is a rapidly growing field of study. Plant root exudates are a conductor for this underground microbial chatter, sending out packages containing amino acids, sugars, hormones, and organic acids, altering microbial biomass and species diversity. These exudates also include “communication chemicals” — secondary metabolites such as fatty acids, sterols, glucosinolates, enzymes, flavanols, lignins, and more. These carbon-based exudates provide diverse benefits and act as payments to stimulate the microbes providing beneficial services. They also act as communication signals to suppress and defend against pathogens and pests as well as playing a key role in aggregate stability and carbon and nitrogen dynamics.
One group of molecules produced by plants and microbes are called volatile organic compounds (VOCs). These VOC can easily move through gas- and water-filled pores. VOC provides multiple services to plants, including triggering plant immunity, promoting plant growth, antimicrobials, food source or attractants.[i] Some microbes produce a huge variety of communication VOCs (over 800 have been identified thus far). Many of the stronger odors you’ll be familiar with are actually microbial VOC’s: feces (indole), urine (ammonia), vomit (butyric), rotting cabbage/farty smells (methyl mercaptan), rotten eggs (hydrogen sulfide gas) and rotten potatoes (dimethyl disulfide). The distinctive “funky” aroma of the mycorrhizal truffle is in part due to dimethyl disulfide and from a molecule called androsterone, a hormone produced by male pigs. This in part explains why pigs can make such good truffle hunters. The fungus sends out this signaling scent to pigs, and to us, to find the truffle when it’s mature. This aids in completing its reproductive cycle, which is limited by sporulating underground. These spores pass through the digestive tract, remaining viable when anything, or anyone, poops in the woods. Geosmin is what gives beetroot its taste and what creates the smell after a shower of rain meets the road on a hot summer’s day. The smell of geosmin released by Streptomyces has been found to attract collembola, (springtails) which then aid in the dispersal of its spores. We can smell geosmin at 5 parts per trillion, a shark can smell blood at one part per million. That means human noses are 200,000X more sensitive to geosmin…than a shark is to blood. [ii]”
Microbes are also streaming chemical and electrical signals to communicate with each other, and with their vital support system, the plants. Testate amoebae, the ones who fashion themselves in hardened shells for protection, have been observed hunting together as a pack, to track down and attack bacteria-feeding nematodes. Their hunting behaviors are determined by these signaling molecules. Even though leaf surfaces are vulnerable to frequent and severe changes in temperature, radiation, and humidity, there are a large variety of microorganisms which thrive on them. Bacteria living on aerial leaf surfaces totally differ from bacteria found in other habitats, including surrounding soil, implying that they have evolved and developed special adaptations to take advantage of leaf surfaces.
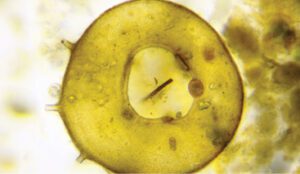
Microscopic view of testate amoeba (Centropyxis aculeata) shell.
The ability to tolerate challenging environments comes through phenotypic (short-term changes in an individual) as well as adaptation (epigenetics). Epigenetic changes passed down through generations of plants, have a role in memory and priming plant defenses to potential pests and diseases. For instance, a study of plants infected by root nematodes, showed an increase in root defense genes in multiple generations. Epigenetics also prepare future generations for extremes of water, nutrient and heat dynamics.[iii](Alonso et al., 2019). It makes me wonder how we might possibly be undermining plant “learning” processes with modern breeding programs, pesticides and fungicides?
Quorum Sensing
Using chemical autoinducers as their “words”, microbes can communicate in multiple languages, both within and between species. In a process called quorum sensing (QS), cell receptors can synchronize their behaviors when sensing specific chemical molecules. Based on the “message” they get, they can either compete or collaborate with other species. These messenger molecules include AHLs (acyl homoserine lactones) and DSF (diffusible signaling factor) to trigger activities at parts per trillion.
The process of QS was first discovered in bio-luminescent bacteria in the 1960’s. With growing concerns around the exploitation of antibiotics, medical research in the ‘90s identified QS’s role in streptococcus virulence. When populations are small, microorganisms are unable to express certain genes as full gene expression requires a community. When numbers increase, autoinducer signals reach a threshold, and the full gambit of gene expression can now switch on and you could end up with a sore throat or a nasty itch. In another process termed quorum quenching, signals are used to inhibit the invasion or growth of another organism. Communication via quorum-sensing is now considered the norm in the bacterial world. Plants also release molecules to suppress QS-specific pathways, including binding molecules, such as terpenes, phenylpropanoids, flavonoid quercetin, sulfur-containing compounds, or quorum quenching molecules; coumarin, vanillic acid, and curcumin.
Communication Signals in Plant Defense
Plants have been in an arms war with diseases and herbivores, both large and small, for hundreds of millions of years. Plants cannot run away from their attackers, so they have developed physical defenses, such as waxy coatings, latex and lignin, as well as a complex system of biochemical and electrical defenses. These signals provide multifaceted surveillance, defense, and detoxification system. They produce a variety of elicitors; proteins (peptides), enzymes, and phytohormones, to defend against pathogens and other invaders. I, along with a growing number of scientists, believe that the future of fungicides and pesticides lies with the biomimicry of these subtle defense systems.
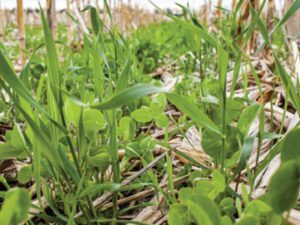
Waxy compounds in the leaves of these healthy clover and ryegrass plants are part of the physical defense system of healthy plants.
A lecturer I heard in 1997 gave a vivid example of plant communication which has stuck with me to this day. When a giraffe browses on the leaves of Acacia trees, the trees respond by releasing puffs of ethylene gas. Acacia downwind respond to this warning signal by making changes in their gene expression that increases the level of tannins in their leaves, which in turn, decreases palatability for other giraffes browsing.
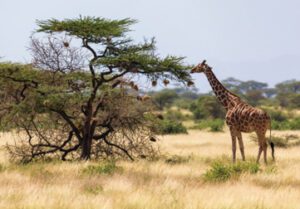
Giraffes browsing the leaves of an acacia tree will trigger a warning signal to other trees and will cause increased tannin production to decrease palatability.
Another example of communication complexity comes from the 1960’s when a former CIA polygraph expert hooked a plant up to his machine and discovered that not only did the plant react to being burned, but it also reacted with a surge of electrical activity whenever he entered the room. A recent study on the “ultrasonic screams” of damaged plants suggests that if farmers can tune equipment to “listen” to plant distress signals, that pesticides and fungicides could be used more precisely with less guess work. Of course, the premise that plants have consciousness has long been hugely controversial, with scientists arguing that without a brain, plants cannot “feel pain”. Plants, however, are very sensitive to their environment, avoiding touch (like crown shyness in trees), responding to light (phototropism), or responding to tactile stimuli (think vines wrapping around a trellis). This touch may be as light as a small raindrop, or the tread from an ant’s toe.[iv]
Plant signals may also provide indirect forms of defense to attract beneficial microbes or predatory/parasitic insects, such as herbivore-induced plant volatiles (HIPVs). Maize plants release a sesquiterpene signal into the root zone when infested by Fusarium, or when attacked by root-feeding beetle larvae, resulting in the enhanced attraction of beneficial nematodes.[v] Plants can sense beneficial predators such as ants, and as a reward for the protection predators and parasites provide, plants increase the quality and volume of extrafloral nectar (EPF). Small signaling molecules and proteins exuded by microorganisms can support the plant in improving overall health and resistance to pathogens and viruses.[vi] The amino acid glutamate, which acts as a neurotransmitter in humans, triggers rapid long-distance signaling using calcium throughout the plant. A study using mustard plants that were genetically modified to fluoresce when cut or munched on by caterpillars showed that plants were able to send internal signals via a “nerve like” process to send a warning of imminent danger. There short video illustrates it well “Plants have their own kind of nervous system.” https://www.youtube.com/watch?v=LeLSyU_iI9o
Two of the most extensively studied mechanisms for plant induced defenses are systemic acquired resistance (SAR) and induced systemic resistance (ISR). After a plant is exposed to microbial elicitors or chemical stimuli, such as chitosan or salicylic acid (SA), the SAR response is activated throughout the plant. Using different mechanisms, ISR relies on signal pathways activated by the hormones jasmonate (JA) and ethylene (ET) and occurs in plants activated by an infection. During ISR, plants respond with a signaling cascade that leads to a broad and long-lasting resistance that is effective against fungi, bacteria, and viruses. Protection by ISR has been reported against a wide range of pathogens, including systemic viruses such as cucumber mosaic virus, root-knot nematodes, leaf-spotting fungal and bacterial pathogens, sclerotinia, crown-rot, stem-blight fungal, damping-off, powdery mildew, Botrytis, take-all, and late blight diseases. As an added bonus, in most cases, the microbes that stimulate ISR also promote plant growth.
What is fascinating is without their beneficial microbial partners, these pathways are inhibited (how amazing is that!) These defense responses are triggered by a range of beneficial microbes including Pseudomonas spp., Trichoderma spp., Mycorrhizae spp., yeasts, Rhizobacteria, Bacillus spp., and other gram-negative bacteria. These microbes trigger plant gene expression, which results in increased growth, immunity, and resistance to stress. Pathogen resistance from trichoderma interactions take multiple forms through the action of its diverse exudates which contain volatile organic compounds (VOC), cell wall degrading enzymes (CWDE), reactive oxygen species (ROS), and antimicrobial secondary metabolites (SM). By forming these interactions, trichoderma increases plant fitness and tolerance against biotic stress either by priming plant defenses, increasing plant growth, or releasing pathogen pressure leading to the enhanced growth response.
Induced Systemic Response. On the left side, the plant is infected with botrytis, a fungal disease, without the presence of beneficial organisms Trichoderma. On the right, ISR from roots colonized by the fungus Trichoderma is primed for response with plant defense hormones jasmonic acid (JA), salicylic acid (SA), and abscisic acid (ABA). [vii] Image based on Martínez-Medina, et al (2013).
What reduces plant and microbial signals?
The ability for plants to communicate with microbiology is influenced by:
- Nutrition
- Plant diversity
- Photosynthetic metabolites
- Plant stress and disturbance
- Plant breeding
- Growth phase
Different species and plants during different growth stages, release a diverse cocktail of root metabolites. Plant stress has a huge influence on a plant’s ability to share resources. Annuals, perennials and early succession plants send different signals depending on their circumstances. Each stage of a plant’s cycle such as actively growing, under attack or ending their life cycle, will trigger a different set of signals to the plant’s environment. Sadly, plant breeding done in altered or sterile environments has altered specific communications from plant roots, so that some cultivars, such as some wheat varieties, no longer signal to beneficial protists for nutrient cycling or bacterial control, or no longer initiate symbioses with mycorrhizae.
Insecticides, fungicides, and herbicides can negatively impact (directly or indirectly) photosynthesis and the subsequent root exudates necessary for quorum signaling. Enzymes and signals involved in nitrogen fixation and transforming free amino acids into complete proteins are compromised by these chemicals.[viii]
Through biopriming seeds with beneficial microbes (compost extracts) or carbon-based foods (humics, seaweeds etc), we can restore microbial communities and enhance plant fitness. Multi-species cover crops, interplanting and diverse rotational crops are key tools in regenerating microbial biomass and diversity.
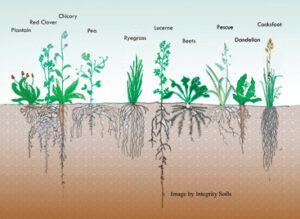
Photo By: Integrity Soils
For the Love of Soil: Strategies to Regenerate Our Food Production Systems translates the often complex and technical know-how of soil into more digestible terms through case studies from regenerative farmers, growers, and ranchers in Australasia and North America which present a compelling testament to the global, rapidly growing soil health movement. Along with sharing key soil health principles and restoration tools, For the Love of Soil provides land managers with an action plan to kickstart their soil resource’s well-being, no matter the scale. n spite of the challenges food producers face, Masters’ book shows that even incredibly degraded landscapes can be regenerated through mimicking natural systems and focusing on the soil first. Order your copy now: www.integrity soils.co.nz
[i] Van Dam NM, Weinhold A, Garbeva P. Calling in the dark: the role of volatiles for communication in the rhizosphere. In: Blande JD, Glinwood R, editors. Deciphering chemical language of plant communication. Springer International Publishing Cham; 2016. p. 175-210.
[ii] www.acsh.org/news/2018/07/28/geosmin-why-we-smell-air-after-storm
[iii] Alonso, C., Ramos-Cruz, D., and Becker, C. (2019). The role of plant epigenetics in biotic interactions. New Phytol. 221, 731–737.
[iv] Nolch, G. (2016). Plants respond to touch. Australasian Science, 37(6), 7.
[v] Van Tol RWHM, Van Der Sommen ATC, Boff MIC, Van Bezooijen J, Sabelis MW, Smits PH. Plants protect their roots by alerting the enemies of grubs. Ecol Lett. 2001; 4:292–4.
[vi] Sofy, A. R., Sofy, M. R., Hmed, A. A., & El-Dougdoug, N. K. (2019). Potential effect of plant growth-promoting rhizobacteria (PGPR) on enhancing protection against viral diseases. In Field Crops: Sustainable Management by PGPR (pp. 411-445). Springer, Cham.
[vii] Martínez-Medina, A., Fernández, I., Sánchez-Guzmán, M. J., Jung, S. C., Pascual, J. A., & Pozo, M. J. (2013). Deciphering the hormonal signaling network behind the systemic resistance induced by Trichoderma harzianum in tomato. Frontiers in Plant Science, 4, 206.
[viii] Tiwari, S., Singh, A., & Prasad, S. M. (2018). Regulation of Pesticide Stress on Metabolic Activities of Plant. In Metabolic Adaptations in Plants During Abiotic Stress (pp. 121-132). CRC Press.
This article first appeared in the 8th Edition of Green Cover's Soil Health Resource Guide.
Also check out the 10th edition, our latest Soil Health Resource Guide, over 90 pages packed with scientific articles and fascinating stories from soil health experts, researchers, farmers, innovators, and more! All as our complimentary gift to you, a fellow soil health enthusiast!